Research
Bacterial pathogens must navigate an ever-changing, deliberately hostile environment to establish and maintain infections. Beneficial commensal bacteria, adapted to the host environment, deplete the available nutrients. The immune system unleashes oxidative, pH, and temperature stress. Antibiotics attack metabolic and replicative processes. Yet pathogens, and the infections they cause, persist due to stress responses activated by extracellular conditions. We study stress signaling and survival in Gram-positive pathogens.
Alarmone signaling in C. difficile
Our group’s main focus is the intestinal pathogen Clostridioides difficile (formerly Clostridium difficile, commonly known as C. diff). This is the most common cause of hospital-acquired infections in the US, and infections have a 20-30% rate of recurrence after treatment. With symptoms ranging from weeks of bloody diarrhea to psuedomembranous colitis to ~30,000 deaths per year, the monetary ($4.8B/year) and human (profound!) costs of C. difficile infections are staggering and the CDC classifies it as an urgent threat. The risk of C. difficile infections is highest in people who have recently taken antibiotics, as a robust community of ‘good’ gut bacteria is the best defense against this pathogen– it is not good at competing for nutrients when its own cell numbers are low. If it does get established during or soon after a course of antibiotics, it becomes very difficult to eradicate.
Nearly all bacterial phyla employ hyperphosphorylated single-base ribonucleotide second messenger molecules to regulate metabolism and cell division. At low levels, these ‘alarmone’ signals slow down biosynethetic pathways to maintain a balance between different metabolic products. When the bacteria sense extracellular stress, like starvation or antibiotic stress, they quickly ramp up alarmone production. If these molecules accumulate above a critical threshold, they shut down cell growth and division and most metabolic processes in order to funnel all of the cell’s resources into transcribing stress response genes. In most pathogens, this includes virulence-related traits like toxin production and expression of antibiotic resistance genes. If a cell survives the crisis, it has to quickly deplete the built-up alarmones to resume normal activity.
Alarmone synthesis and degradation have been extensively studied. Conserved synthetase domains transfer a pyrophosphate from an ATP donor to the 3’OH of a GTP/GDP/GMP substrate to make a pppGpp/ppGpp/pGpp alarmone product. A substrate with (n) phosphates will yield a product with (n+2) phosphates. When conditions improve, hydrolase domains remove a pyrophosphate from the 3′ end of the alarmone, and the original substrate is regenerated. The synthetase and hydrolase domains are so well conserved that they haven’t attracted much notice as antibiotic targets– anything that affected them would be really broad-spectrum, harming helpful bacteria as well as pathogens.
HOWEVER, this highly conserved pathway manifests a little bit differently in C. difficile. This organism encodes two highly conserved synthetase genes, and disruption of alarmone signaling through RNA interference or with chemical inhibitors increases its antibiotic susceptibility. However, they only use GTP and GDP as substrates, but exclusively generate the triphosphate alarmone pGpp as an alarmone product. C. difficile needs to cleave a 5′ phosphate bond on its guanosine substrate in order to form a 3′ phosphate bond.
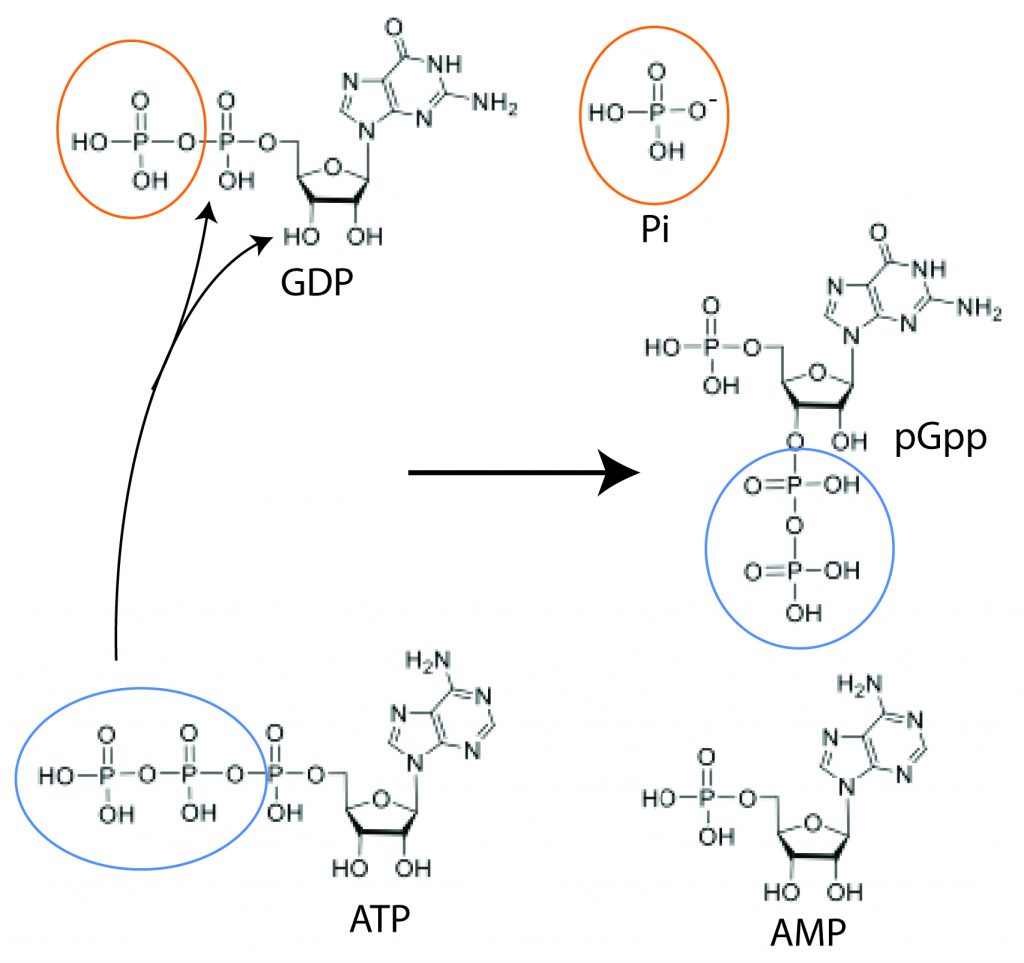
This unusual biochemistry might be an opportunity– if we can identify the positions in the C. difficile synthetase active sites that contribute to the differences between the clostridial enzymes and its homologs in other organisms, we might be able to design alarmone synthesis inhibitors that are specific to C. difficile. Such inhibitors could be given as adjuvants to antibiotic treatment, to boost the efficacy of the drugs against C. difficile alone, sparing commensal bacteria. There are a lot of questions to answer before we get to that point.
What residues in the clostridial enzymes contribute to their unique biochemistry?
Can targeted mutation allow C. difficile synthetases to make larger alarmones, or force homologs from other organisms to cleave guanosine 5′ phosphate bonds to make small ones?
How does C. difficile respond to the larger alarmones produced by other bacteria?
Can it sense them? Does using the smaller alarmone for its internal regulation allow it to ‘eavesdrop’ on surrounding bacteria by perceiving their stress signals?